Dietary Essential Fatty Acids and Reproduction in Dairy Cows
Feeding of fat to dairy cows enables a more energy-dense feedstuff to be provided in the ration, say researchers B. Jones, A. Bowen, M. Martin, and R. Ax. This feature was taken from the proceedings of the High Plains Dairy Conference. A full link to the proceedings is provided below.Introduction
Several excellent reviews have been written, which substantiate the merits of feeding fat. In recent years, omega-6 (linoleic acid) and omega-3 (linolenic acid) fatty acids have received attention because they are classified as essential fatty acids (EFA) for dairy cows. Being categorized as an EFA means that feeding of these fats does not result in them remaining patent for absorption across the intestinal wall. Actually, the rumen microbes biohydrogenate both of these EFA, eliminating their double bonds, converting them to stearic acid.
To restrict biohydrogenation, EFA can be fed as long chain calcium salts, so they escape rumen conversion to stearic acid. They arrive at the intestine in their native form, are absorbed, and then delivered through the circulation to virtually every tissue in the body. To that end, research trials are being conducted to delineate specific roles for EFA in terms of hormone production, tissue repair and function, immune function, and embryonic development. The purpose of this article is to summarize our current understanding of the importance of EFA to reproductive events in the postpartum (PP) cow.
Mechanisms of EFA Action
Polyunsaturated fatty acids (PUFA) are precursors to cholesterol, which is an antecedent to steroid hormones. The corpus luteum (CL) uses cholesterol to make progesterone (P4), and P4 is itself a precursor to estradiol (E), but it is also an inhibitor of E secretion. Linoleic acid (along with its elongated product, eicosapentanoic acid) is a proven inhibitor of cyclooxygenase in endometrial tissues (Haag, 2001). Consequently, endometrial secretion of the prostaglandin (PG) PGF2α from the uterus can be suppressed. This action might also be aided by the effect fat has in repressing E17β secretion (Staples et al., 1998); thereby reducing PGF2α secretion and decreasing the sensitivity of the CL to PGF2α. This helps prolong the life and functionality of the CL and allows it to increase P4 concentrations. Higher pre-breeding concentrations of P4 have a positive impact on conception rates, discussed subsequently. A brief pathway of steroid hormone synthesis is shown in Figure 1. If PUFA are limited in supply, the chain of events shown in Figure 2 is likely.
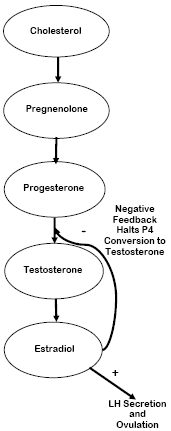
Synthesis of Eicosanoids
Eicosanoids are biological signaling factors, some of which are PG. Prostaglandins are often used exogenously by producers to treat reproductive problems and for synchronization of the estrous cycle to set up timed AI. Some of the general functions of the various classes of PG include: causing ovulation, resuming cyclicity, enhancing visible signs of estrus, and increasing blood flow to the ovaries to promote follicle growth (Senger, 2005). The most commonly known PG is PGF2α, which is responsible for the regression of the CL. Prostaglandin E is another common prostaglandin, and is thought to aid in initiating ovulation and thus the estrous cycle.
Progesterone (P4)
Progesterone is commonly referred to as the pregnancy hormone; as increased concentrations of P4 are maintained following conception, the higher the likelihood the animal will remain pregnant. Additionally, it is believed cattle with higher concentrations of P4 at the end of the estrous cycle show stronger signs of estrus (Staples et al., 1998). Stronger signs of estrus lead to more accurate estrous detection and correctly timed breeding. Fonseca and co-workers (1983) found that higher pre-breeding P4 concentrations lead to higher conception rates. The conception rate was increased 12.4 % for each ng/mL serum P4 concentration on d 12 of the cycle prior to AI. Shemesh et al. (1983) confirmed the findings of Fonseca et al. (1983).
Synopsis of Staples, Burke and Thatcher
Staples and colleagues (1998) reviewed over 100 studies and compiled the results into one paper on “Optimizing energy nutrition for reproducing dairy cows.” Throughout the review, previous studies were grouped together to illustrate the effects of EFA on reproductive events. Ultrasound was used in 6 studies to determine the follicle sizes 1 d prior to ovulation; however, days PP among cows varied greatly both within and between studies. An early PP cow likely will have smaller follicles than a cow 100 d fresh, and comparing such studies, and making comparisons within study, becomes statistically questionable. Currently, there is no reproductive equivalency test (compared to mature equivalent for comparing heifers to multiparous cows for milk production) that will determine when a cow resumed cyclicity or how many cycles she has had based upon a single ultrasound on a randomly chosen day PP.
Examining the mean of the diameter of dominant follicles, it seemed clear that supplemental fats were improving follicular sizes. Yet, simply averaging the diameter of dominant follicles across those 5 studies was unreasonable; because, as aforesaid, there is no reproductive equivalency test available. In some of the studies PGF2α was used as part of a synchronization regimen, and the follicles were measured before AI. In others, no synchronization regimen was utilized and the follicles were measured prior to AI, the timing of which was dependent on the opinion of the herd manager. Days PP varied from 38 to over 100 within and between studies. This type of comparison further emphasizes the need for consistency when studying the effects of nutrition on reproduction.
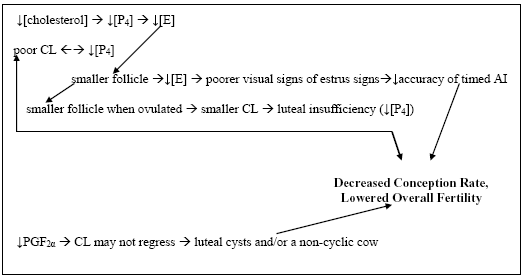
Staples and coworkers also state “11 studies that reported an improvement (P < 0.10 or > 15 percentage unit difference between means) either in first AI service conception rate or in the overall rate of conception or pregnancy” had fed supplemental EFA. Five of those studies fed calcium salts of long chain fatty acids (Ca-LCFA). Again, compiling data from 5 studies, cows fed Ca-LCFA had a 5.8 % increase in signs of standing estrus, 5.9 % increase in the activity of the ovaries, and 12 % more cows responded to a 20 mg dose of PGF2α (normal dose is 25 mg). While it is safe to conclude there is a positive influence of feeding Ca-LCFA on reproduction, these percentages can be misleading. The breeding programs utilized in each of the 5 studies varied once again. For example, in Scott et al. (1995) breeding ranged from 38 to 90 d in milk (DIM). The study was conducted across 5 separate herds and the initiation of breeding was left to the herd manager’s discretion. Sklan et al. (1991) allowed artificial insemination if the cow showed signs of estrus after 58 DIM. This re-confirms the importance of maintaining a consistent breeding interval across treatments to obtain objective fertility data.
While Staples and cohorts found 11 studies elucidating positive effects of supplemental EFA on reproduction, it is of note that 3 studies reported a strong negative influence of feeding fat on conception rate at first AI service. However, in each case a lower conception rate was accompanied by a large increase in milk production. High milk yield has frequently been linked to lowered fertility in lactating cows, but is inferior to NEB as an influencer of reproductive performance (Domecq et al., 1997).
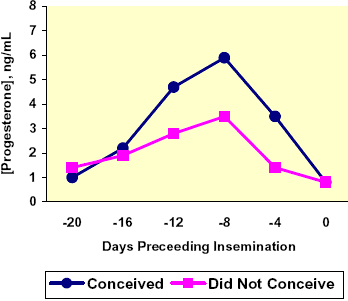
From d 7 PP until d 21 to d 25 PP the uterus is the site of a highly concentrated immune response. The uterus is a sterile environment throughout gestation and is exposed to pathogenic and environmental microorganisms at parturition, which must be neutralized before the health and function of the organ can be restored (Senger, 2005). This process can be described as 3 distinct processes (cellular immune response, a uterine endocrine response, and an ovarian endocrine response) as follows (Jainudeen and Hafez, 2000):
Table 1. Results of 5 studies summarized by Staples et al. (1998).
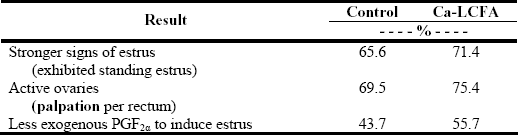
- A defense mechanism that includes a massive infiltration of lymphocytes into the uterine lumen to phagocytize the major pathogens present in the early PP period.
- A massive release of PG during the first 2 wk PP induces myometrial contractions and evacuation of fluids and fetal membrane debris from the uterus.
- Estrogen secreted by the ovaries before the uterus becomes more resistant to infection after the first ovulation.
With respect to the cellular immune response, the cells which are most abundant and commonly detected during this period are polymorphonuclear leukocytes (PMN), often referred to as neutrophils. Polymorphonuclear leukocytes play a primary role immediately PP for the breakdown of fetal/maternal attachment at the placentome, allowing fetal membranes and spent caruncular tissue to be shed and expelled with locia (LeBlanc, 2006). This response is necessary and integral to the timely and complete recovery of the uterus. The uterine inflammatory response during parturition releases chemoattractant factors causing increased vascular permeability and induces diapodesis of circulating PMN into the uterus (Lu and Rosenbusch, 2004). Immunoglobulin G (IgG) is found in greater proportion to other immunoglobulins within the reproductive tract when compared to other tissues of the body (Corbeil et al., 1976). The presence of complement in conjunction with IgG and the small native population of PMN PP aid in recruitment of circulatory PMN as well as other leukocytes and in coordinating the localized immune response.
By actively increasing immune cell presence based upon physiological changes in response to estrogens, the female reproductive tract preemptively defends itself against infection. The presence of native and recruited populations of circulating PMN results in a rapid and indiscriminate clearing of extraneous sperm, bacteria, viruses, and any other foreign cellular debris introduced into the tract from the time of insemination until the conceptus enters the uterus (Tomlinson et al., 1992; Hammon et al., 2006).
The reproductive tract as well as the immune system utilizes EFA for signaling, chemical markers, and precursors for enzyme and hormone activity. In the uterus the function of EFA results from the incorporation of these fats into endometrial cell membranes for conversion to PG.
In addition to the endogenous organs of the female reproductive tract, the placenta (a functional organ in and of itself throughout gestation) has been shown to preferentially integrate EFA into cell membranes increasing activity and efficiency of several metabolic pathways; altering the hormone profiles produced by the fetal membranes as well as contributing to the decreased incidence of retained fetal membranes PP (Bilby et al., 2006a,b; Wischeral et al., 2001). The mechanism for which the reduced incidence of retained fetal membranes occurs is greatly unknown; although evidence that PMN, which are integral to the shedding of caruncular tissue and breakdown of fetal placentomes for the purpose of expulsion, is related to E17β concentrations and the preferential use of linoleic acid by PMN. Both phenomena are directly related to the concentration and proportion of EFA in the diet, which are successfully incorporated into cellular membranes (Wischeral et al., 2001)
Importance of EFA Early Postpartum
Research at The University of Arizona has centered on evaluating the role of EFA in the early PP interval. Since EFA are precursors for steroids and PG, they should be related to re-initiation of cyclicity PP. Data in Figure 4 confirm that EFA directly affects postpartum reproductive events. Holstein cows were fed 0.11 kg/hd/d of Megalac® (Arm & Hammer Animal Nutrition, Princeton, NJ) 21 d prepartum, then received 0.16 kg/hd/d of Megalac® or Megalac®-R through the voluntary waiting period. Megalac®-R contains 4.5 fold the amount of linoleic acid and 23.5 fold the amount of linolenic acid compared to Megalac®. Those extra fats in Megalac®-R doubled the incidence of ovulations by 30 DIM, contributed to almost 1 additional cycle by 60 DIM, and markedly improved uterine health by 60 DIM compared to cows fed Megalac® (Figure 4). All of those physiological responses have been reported to reduce services per conception and days open in previous studies. A point to consider here: many people are extending voluntary waiting periods with timed A.I. programs. If uterine health and cyclicity are hastened by feeding EFA, then timed A.I. should occur earlier postpartum to capitalize upon the physiological advantage of cows being ready for breeding earlier.
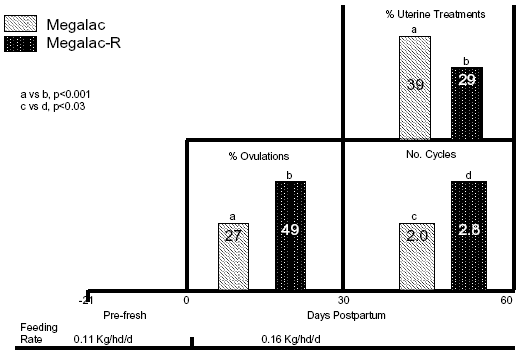
Role of EFA in Pregnancy Recognition and Maintenance
Pregnancy recognition in the bovine occurs between d 15 and 18 post-estrus with the conceptus maintaining the CL of pregnancy. Not unexpectedly, then, it is estimated that 40 % of embryonic death loss occurs during d 8-17 post-estrus and could largely be a result of inability to maintain the CL. This is accomplished through the production of interferon-tau (INF) which inhibits the ability of the uterine endometrium to produce pulsatile PGF2α which is responsible for the degradation of luteal tissue (Thatcher et al., 2001). There is evidence indicating that the length of the conceptus is directly related to the production capacity of INF (Bilby et al., 2006a). Of equal importance is the observation that inclusion of n-3 fats in the diet via fish oil or Ca-LCFA increases the length of the conceptus, likely improving the ability to synthesize INF and enhancing the likelihood that pregnancy recognition through maintenance of the CL will occur (Bilby et al., 2006a). Other observed effects of feeding n-3 fats were through modulation of gene expression of P4 receptors, estrogen receptors, and PGF2α precursors (Bilby et al., 2006b).
Conclusions
Feeding supplemental fat to dairy cows no longer has energy density as a primary consideration in the ration. Essential fatty acids, notably linoleic (omega-6) and linolenic (omega-3) acids have direct effects on physiological processes such as cellular membrane integrity, hormonal pathways, and immune function. Clearly, they are important to uterine health, and that is accompanied by earlier ovulations postpartum and more cycles during the voluntary waiting period. These events lead to improved fertility earlier in the postpartum interval, because they reduce services per conception and days open.
Further Reading
![]() |
- | You can view the full conference proceedings by clicking here. |
August 2008